Protozoa Locomotory Systems
Most free-living protozoans move, using either cilia, flagella, or flowing cytoplasmic fingers called pseudopodia ( pseudo = G: false; podium = G: foot). The members of some species possess different locomotory systems at different stages of the life cycle or under different environmental conditions. it has become all too clear that similar locomotory systems among different protozoan groups do not always indicate close evolutionary connections.
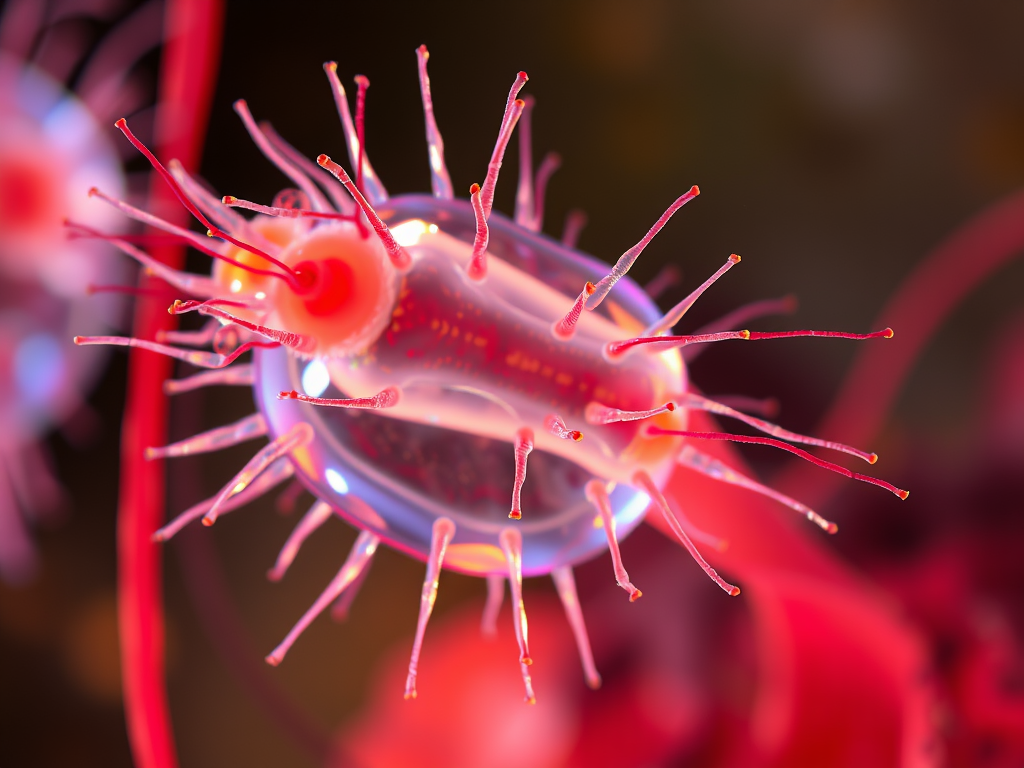
Structure and Function of Cilia
The ultrastructure of cilia is remarkably uniform among all organisms in which cilia occur, from protozoans to vertebrates, probably reflecting evolution by common descent. Although modifications to the basic plan are found sporadically, cilia are always cylindrical, with each cilium arising from a basal body (kinetosome). Within the cilium are a number of long fibers called microtubules, composed of a protein known as tubulin. Tubulin is extremely similar to the actin of metazoan striated muscle.
A cross section of a cilium’s kinetosome in the region beneath the outer body surface shows a ring of 9 groups of microtubules, with 3 microtubules to a group ( lower right). The A, or innermost microtubule of each group, is physically connected to the C, or outermost microtubule of an adjacent group, via a thin filament. Additional filaments connect the A microtubule to a central tubule, like the spokes of a wheel. This configuration of microtubules changes somewhat near the distal end of the cilium.
A cross section through a cilium external to the body surface shows a ring of nine groups of microtubules, with only two microtubules per group; the C microtubule is not found ( upper right). Instead, a pair of dynein arms projects outward from each A microtubule toward the B tubule of the neighboring pair of microtubules. The primary protein component of these arms (dynein) is similar in some respects to muscle myosin. Like myosin, dynein possesses the ability to cleave adenosine triphosphate (ATP), releasing chemical energy.
One pair of microtubules is located centrally within the cilium. These two microtubules form the central shaft of the cilium, and they are often surrounded by a membrane constituting a central sheath. Distinct filaments extend from the A microtubule of each outer doublet in toward this central sheath. The entire microtubular complex, consisting of the nine doublet microtubules and the inner pair of single microtubules, is termed the axoneme.
Although many details of ciliary operation remain to be elucidated, present evidence indicates that ciliary bending is achieved through the differential sliding of some groups of adjacent microtubules relative to others within the same cilium. The energy for this sliding appears to derive from the ATPase activity of the dynein arms, and the sliding itself appears to involve an interaction between tubulin and dynein, in a manner highly reminiscent of the interaction between the actin and myosin filaments of metazoan striated muscle. As we will see later in this chapter, microtubules play a variety of roles, both direct and indirect, in the locomotion of most cells—even in those lacking cilia.
The rhythmic and coordinated bending and recovery of cilia enable both locomotion and food collection. Ciliates are the fastest-moving of all protozoans, achieving speeds of up to 2 mm per second. In addition, ciliary activity is probably important in ridding the surroundings of wastes and in continually bringing oxygenated water into contact with the general body surface.
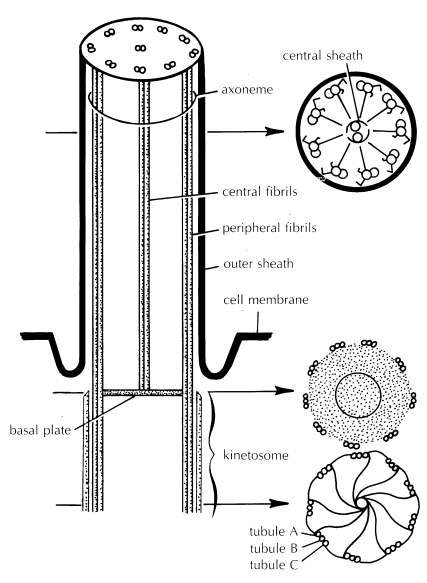
To be effective—that is, to create unidirectional movement of an organism in water or unidirectional flow of water past an organism—the power stroke and the recovery stroke of a cilium must differ in form. By analogy, if you are swimming the breaststroke and move your arm forward to the front of your head by the same path and with the same force that you used to bring the arm back to your side, you will move backward by about the same distance that you moved forward during your power stroke.
The cilium faces a similar dilemma. During ciliary locomotion, the power stroke, as the name implies, works against the environment. The cilium is outstretched for maximum resistance as it bends downward toward the body. During the recovery stroke, the cilium bends in such a manner as to considerably reduce resistance, doing less work against the environment.
Thus, the recovery stroke does not undo the work of the power stroke. In addition, the cilium moves fastest in the power stroke, increasing the force of the power stroke relative to that of the recovery stroke. By analogy, more force is required to move your arm through the water quickly than slowly.
Structure and Function of Flagella
Flagella are structurally much like cilia. In cross section, flagella exhibit the same characteristic arrangement of nine pairs of microtubules ringing a pair of central microtubules over most of their length. Like cilia, flagella are produced from basal bodies, and the movement of flagella is believed to involve the sliding of microtubules in relation to each other.
The cross-sectional appearance of the basal body of a flagellum resembles that previously described for cilia. Indeed, the structural and functional similarities between cilia and flagella are so striking—and differ so much from those of bacterial flagella—that eukaryote cilia and flagella are sometimes grouped together under the descriptive heading undulipodia.
Unlike cilia, however, flagella often bear numerous, external hairlike projections (mastigonemes) along their length. Presumably, these mastigonemes increase the effective surface area of the flagellum, thus increasing the power that it can generate when it moves through the water. Flagella are usually longer than cilia, and the typical flagellate bears many fewer flagella than the typical ciliate has cilia.
Unlike with cilia, several waves of movement may be in progress simultaneously along a single flagellum, and the wave may be initiated at the tip of the flagellum rather than at the base, pulling the organism forward. Flagellate locomotion can be fairly rapid, up to about 200 μm per second. This is only about one-tenth the speed attained by many ciliates but is about 40 times that reached by the fastest amoebae, which typically move using pseudopodia
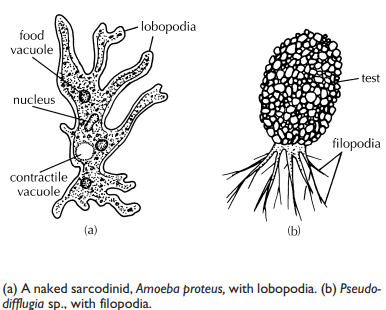
Structure and Function of Pseudopodia
Pseudopodia are characteristic of amoebae. They are used both in feeding and locomotion 5 and come in several forms, Lobopodia are typical of the familiar amoeba; they are broad with rounded tips, like fingers ( lobo = G: a lobe), and bear a distinctly clear ectoplasmic area, called the hyaline cap, near each tip. In contrast, filopodia ( filo = L: a thread) lack a hyaline cap, are very slender, and often branch. With both types, the organism flows into the advancing pseudopodium, a process called cytoplasmic streaming.
The amoeboid body is thus formless, lacking permanent anterior, posterior, or lateral surfaces; pseudopodia can generally form at practically any point on the body surface. This is also true of parasitic amoeboid species, including the ubiquitous agent of amoebic dysentery, Entamoeba histolytica.
The mechanism by which pseudopodia form and change shape is not certain, although it seems clear that movement involves a controlled transition of cytoplasm between the gelatinous, ectoplasmic form (gel) and the more fluid, endoplasmic form (sol). We don’t fully understand the factors that coordinate this transformation in different parts of the cell, although the hypotheses have become wonderfully complex during the past decade. The transformation may involve the interaction of actin and myosin molecules, both abundant in amoeba cytoplasm.
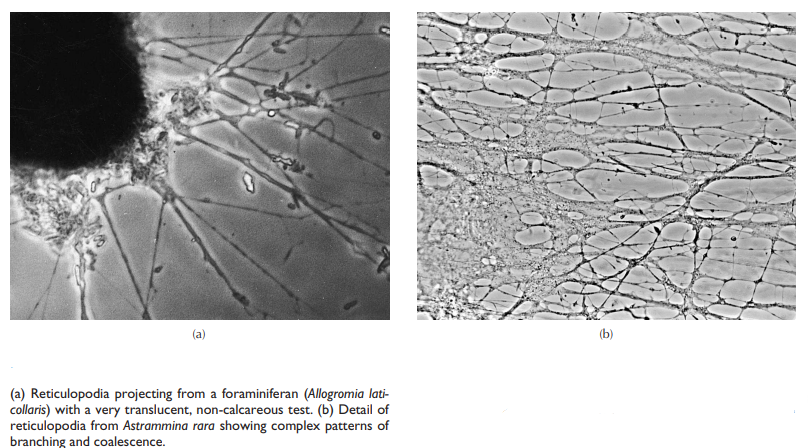
A model of sliding actin filaments has been proposed, in which actin and myosin interact in a way that resembles their interaction in the muscle tissue of multicellular animals. Other hypotheses minimize the role of myosin in the gel-sol-gel transitions and instead emphasize the potential role of selective actin polymerization and depolymerization. In one model, localized actin disassembly creates an area of increased osmotic pressure, which draws water from the more central region of the body to the periphery, forming a pseudopod. The actin is then repolymerized to form a bracing network, fixing the pseudopod’s shape until the next bout of depolymerization.
Whatever the mechanism, pseudopodial locomotion is extremely slow, usually less than 300 μm per minute (1.8 cm per h). Pseudopodia can also come in very elaborate, anastomosing forms called reticulopodia ( reticularia = L: a network), in which extremely thin filaments branch and coalesce repeatedly in highly complex patterns and exhibit a characteristic and striking bidirectional streaming; reticulopodia are especially characteristic of foraminiferans, Finally, some pseudopodia radiate outward along thin spines, or along equally thin rods composed of microtubules. These axopodia ( axo = G: an axle; podia = G: foot) are used more for food collection than for locomotion
Discover more from ZOOLOGYTALKS
Subscribe to get the latest posts sent to your email.