INTRODUCTION
Molecular analysis using Fluorescence spectroscopy is a powerful technique used in biology to study the properties of biomolecules that exhibit fluorescence. Fluorescence is a phenomenon in which a molecule absorbs light of a particular wavelength and re-emits light at a longer wavelength. The emitted light is often of a lower energy and longer wavelength than the absorbed light.
In biology, fluorescent molecules such as proteins, dyes, and probes are used to label and visualize cellular structures and biomolecules. Fluorescence spectroscopy can provide information on the structure, conformation, dynamics, and interactions of these molecules.
One common application of fluorescence spectroscopy is in the study of protein-protein interactions, which is crucial for understanding various biological processes such as signal transduction, enzymatic reactions, and gene regulation. By using fluorescently labeled proteins, researchers can monitor the binding and dissociation of proteins in real-time, providing insights into the kinetics and thermodynamics of protein-protein interactions.
Fluorescence spectroscopy is also used in the study of cellular processes such as ion transport, membrane potential, and intracellular signaling. By using fluorescent probes, researchers can monitor changes in cellular activity and localization of molecules within cells.
PRINCIPLE
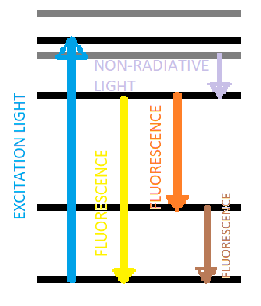
Fluorescence spectroscopy is based on the principle of the absorption of light by a molecule, which results in the excitation of electrons to higher energy levels. When the excited electrons return to their ground state, they release energy in the form of light, which is referred to as fluorescence. The wavelength of the emitted light is longer than the wavelength of the absorbed light, and it is characteristic of the molecule and its environment.
The intensity of fluorescence is proportional to the concentration of the fluorescent molecule, the efficiency of the excitation process, and the quantum yield of the fluorescence. The quantum yield is the ratio of the number of photons emitted to the number of photons absorbed, and it depends on the properties of the molecule and the environment.
The fluorescence spectra of molecules typically exhibit a broad emission band with a maximum at a characteristic wavelength, which can be measured using a fluorescence spectrometer. The excitation spectra of molecules, which show the dependence of the fluorescence intensity on the excitation wavelength, can also be measured.
The wavelength of the excitation and emission light is important for fluorescence spectroscopy, as different fluorescent molecules have different absorption and emission spectra. This allows researchers to selectively excite and detect specific molecules within a complex sample. For example, fluorescently labeled antibodies can be used to specifically target and detect proteins within a cell.
- FLUORESCENCE SPECTRA: Fluorescence spectra are graphs that plot the intensity of the emitted fluorescence as a function of the emission wavelength. The fluorescence spectra provide information on the emission properties of the fluorescent molecule, including its peak emission wavelength, intensity, and shape. By analyzing the fluorescence spectrum, researchers can obtain information on the electronic and structural properties of the fluorescent molecule, such as its excitation and emission energies, quantum yield, and Stokes shift. Fluorescence spectra are used in a variety of applications, including the characterization of fluorescent probes, monitoring of biochemical reactions, and imaging of live cells and tissues. The fluorescence spectrum can be obtained using a fluorescence spectrophotometer, which measures the intensity of the emitted fluorescence at different emission wavelengths.
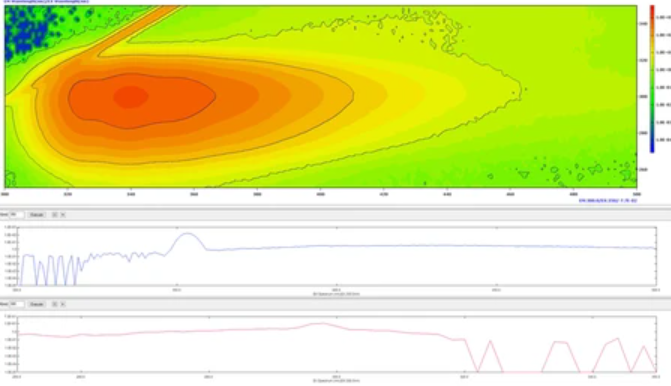
FORMULA
The mathematical expression for fluorescence intensity in fluorescence spectroscopy is
I = ε x c x φ x η,
where ε is the molar extinction coefficient of the fluorescent molecule, c is the concentration of the fluorescent molecule, φ is the quantum yield, and η is the detection efficiency of the measurement system. The fluorescence intensity can be used to obtain information on the concentration, quantum yield, and extinction coefficient of the fluorescent molecule. By measuring the fluorescence intensity as a function of the excitation wavelength, researchers can obtain a fluorescence spectrum that provides valuable information on the properties of the fluorescent molecule.
WORKING
In a biological sense, fluorescence spectroscopy is commonly used to study the properties of fluorescent molecules or fluorescently labeled biomolecules in solution or within living cells.
The fluorescence spectrophotometer is used to excite the fluorescent molecule or probe with a specific wavelength of light, and the emitted fluorescence is then detected and measured. The fluorescence emission spectrum is recorded and analyzed to obtain information on the properties of the fluorescent molecule or probe, such as its concentration, fluorescence intensity, and lifetime.
Fluorescence spectroscopy can be used to study a wide range of biological processes, including protein folding, enzyme activity, protein-protein interactions, and cellular signaling. It can also be used for imaging of biological samples, such as fluorescently labeled cells or tissues, providing information on the distribution and localization of the labeled biomolecules.
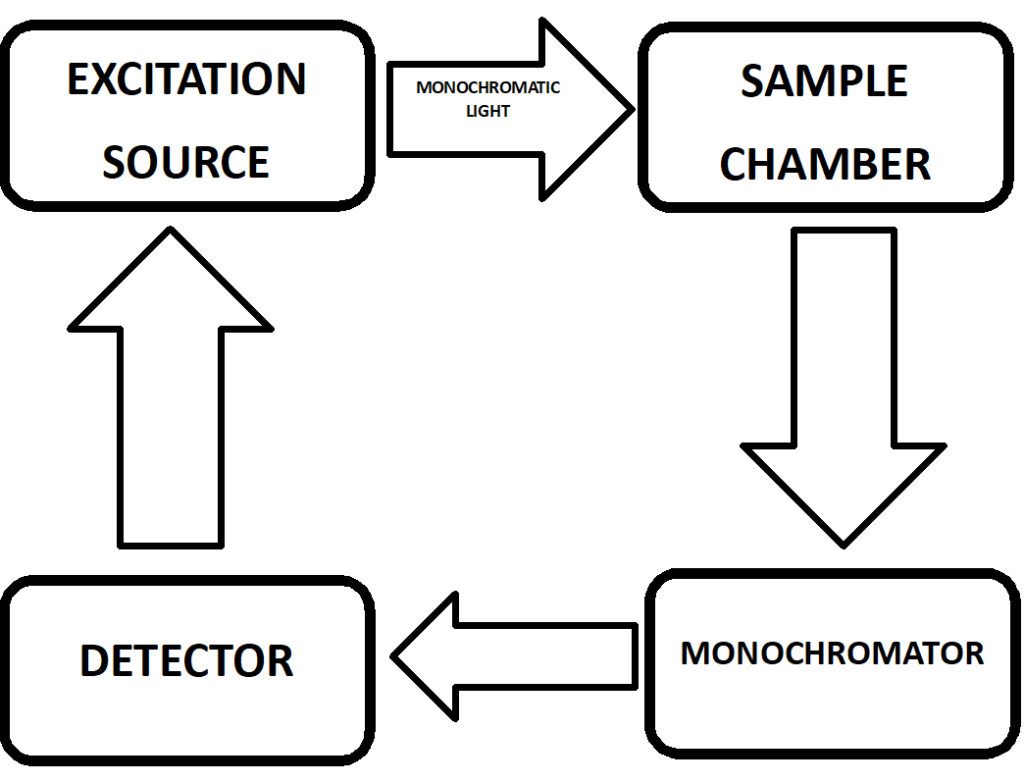
FLUORESCENCE PROBES
- Fluorescein: a small fluorescent dye that is commonly used in a variety of applications, including labeling proteins and nucleic acids, and detecting reactive oxygen species.
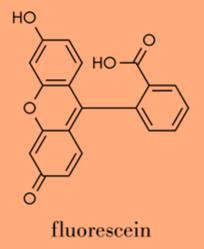
- Green fluorescent protein (GFP): a genetically-encoded fluorescent protein that is widely used as a reporter in live cell imaging studies. GFP can be expressed in cells and organisms to label specific structures or proteins of interest.
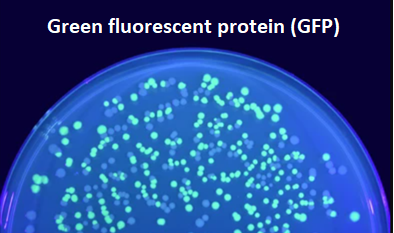
- Rhodamine B isothiocyanate (RBITC): a fluorescent dye that is used to label antibodies and other biomolecules for detection in immunoassays and fluorescence microscopy.
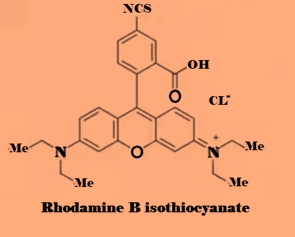
- Quantum dots: nanoparticles that exhibit unique fluorescence properties due to their small size and high surface-to-volume ratio. Quantum dots can be used for a variety of applications, including cellular imaging, drug delivery, and biosensing.
- BODIPY (boron-dipyrromethene) dyes: a class of highly fluorescent dyes that are used for labeling lipids, nucleic acids, and proteins.
INSTRUMENTATION
Fluorescence spectroscopy instrumentation typically consists of the following components:
- Light source: A high-energy light source, such as a xenon or mercury lamp, is used to provide the excitation light.
- Monochromator: The monochromator is used to select a specific excitation wavelength and emission wavelength for the fluorescence measurements. It can also be used to adjust the bandpass of the excitation and emission light, which affects the sensitivity and resolution of the measurement.
- Sample holder: The sample holder holds the sample to be measured and typically includes a cuvette or a sample stage for imaging applications.
- Detector: The detector measures the emitted fluorescence from the sample and converts it into an electrical signal. Photomultiplier tubes (PMTs) are commonly used as detectors in fluorescence spectroscopy due to their high sensitivity and fast response time.
- Data acquisition system: The data acquisition system records the emitted fluorescence signal as a function of wavelength and generates a fluorescence spectrum.
Modern fluorescence spectrophotometers may also include features such as automated sample handling, temperature control, and advanced data analysis software.
TYPES
There are several types of fluorescence spectroscopy that are commonly used in biological research, including:
- Steady-state fluorescence spectroscopy: This is the most common type of fluorescence spectroscopy, which measures the intensity of the fluorescence emission at a single wavelength following excitation. It is used for determining the concentration of fluorescent molecules or probes, and for studying the spectral properties of fluorescent molecules.
- Time-resolved fluorescence spectroscopy: This type of fluorescence spectroscopy measures the decay of the fluorescence emission over time following excitation. It is used for studying the kinetics of biochemical reactions, the interactions between biomolecules, and the conformational changes of proteins.
- Fluorescence polarization spectroscopy: This measures the degree of polarization of the emitted fluorescence, which is related to the mobility and orientation of the fluorescent molecule or probe. It is used for studying the structure and dynamics of biomolecules and membranes.
- Fluorescence correlation spectroscopy: This measures the fluctuations in the intensity of the emitted fluorescence over time, which are related to the diffusion of fluorescent molecules or probes. It is used for studying the dynamics of biomolecules and the transport of molecules within cells.
APPLICATION IN BIOLOGY
Fluorescence spectroscopy has numerous applications in biology, as it allows researchers to study the properties of fluorescent molecules and their interactions with biological systems. Some common applications of fluorescence spectroscopy in biology include:
- Protein structure and function: Fluorescence spectroscopy can be used to study the conformational changes and folding of proteins, as well as their interactions with ligands, other proteins, and membranes. It is also used to measure enzyme kinetics and activity.
- Cellular imaging: Fluorescence microscopy allows for the visualization and tracking of fluorescently labeled molecules and cells in living tissues. This technique has a wide range of applications, including studying cellular trafficking, cell signaling, and gene expression.
- Molecular interactions: Fluorescence spectroscopy can be used to study the interactions between biomolecules, including protein-protein interactions, protein-DNA interactions, and protein-ligand interactions. This can provide insights into the mechanisms underlying complex biological processes such as signal transduction, gene regulation, and disease pathology.
- Drug discovery and development: Fluorescence spectroscopy is used in drug discovery to screen for compounds that interact with target proteins or biomolecules, and to optimize their binding properties. It is also used to study the pharmacokinetics and biodistribution of drugs in living organisms.
- Environmental monitoring: Fluorescence spectroscopy can be used to monitor pollutants and contaminants in the environment, including water, air, and soil. It is also used to study the effects of environmental stressors on organisms and ecosystems.
CONCLUSION
Fluorescence spectroscopy is a powerful tool in biological research, providing a non-invasive and highly sensitive method for studying the properties and interactions of fluorescent molecules and probes. Its versatility allows for a wide range of applications, from studying protein structure and function, to cellular imaging, molecular interactions, drug discovery, and environmental monitoring. The development of new fluorescent probes and advanced instrumentation has expanded the scope of fluorescence spectroscopy, enabling researchers to obtain more detailed information on biological processes at the molecular level. As a result, fluorescence spectroscopy is an essential technique in modern biological research, with the potential to contribute to advances in fields such as medicine, biotechnology, and environmental science.
Discover more from ZOOLOGYTALKS
Subscribe to get the latest posts sent to your email.